Projects
Explicit Algebraic SGS modelling for large-eddy simulations (LES)
The EAM is based on algebraic approximations of the modelled transport equations of SGS stresses and aims to better describe SGS anisotropy, which is strong near the walls, than standard eddy viscosity models through a more complete description of the SGS physics. The main formulation of the subgrid-scale (SGS) Reynolds stress, described in detail in (Marstorp et al., JFM, 2009) is given below:

For different friction Reynolds numbers (550, 2000 and 5200), the use of the EAM allows for a reduction of computational resources in a pseudo-spectral code (Chevalier et al., KTH, 2007)(Montecchia et al., PRF 2017) .
In OpenFOAM, the use of EAM together with the reduction of Rhie and Chow interpolation, was shown to give substantial improvements in turbulent channel flows for the prediction of the skin friction and the mean velocity profile, compared to dynamic Smagorinsky model (DSM) (Germano et al., PoF 1991; Lilly et al., PoF 1991). Some improvements were also found for other statistics such as the individual Reynolds stresses. The mean velocity prediction improvement can be translated to roughly a 50% reduction in the requirement for number of grid points to achieve a given degree of accuracy. Moreover, the model behaviour and grid dependency were found to be fairly consistent with previous results using a spectral discretisation. However, the resolution requirement is 2-3 times higher for this typical second order finite volume method, compared to a spectral method.
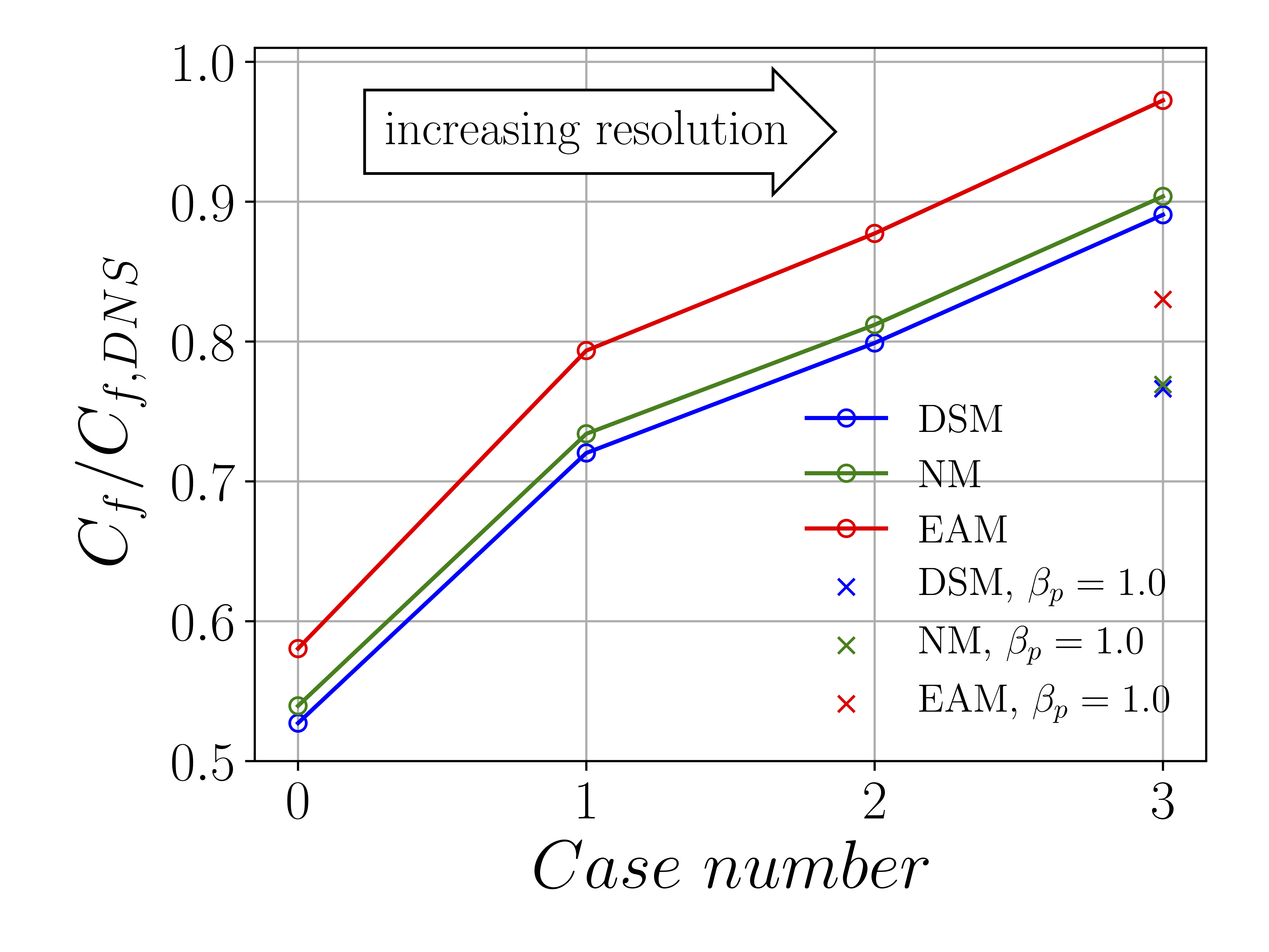
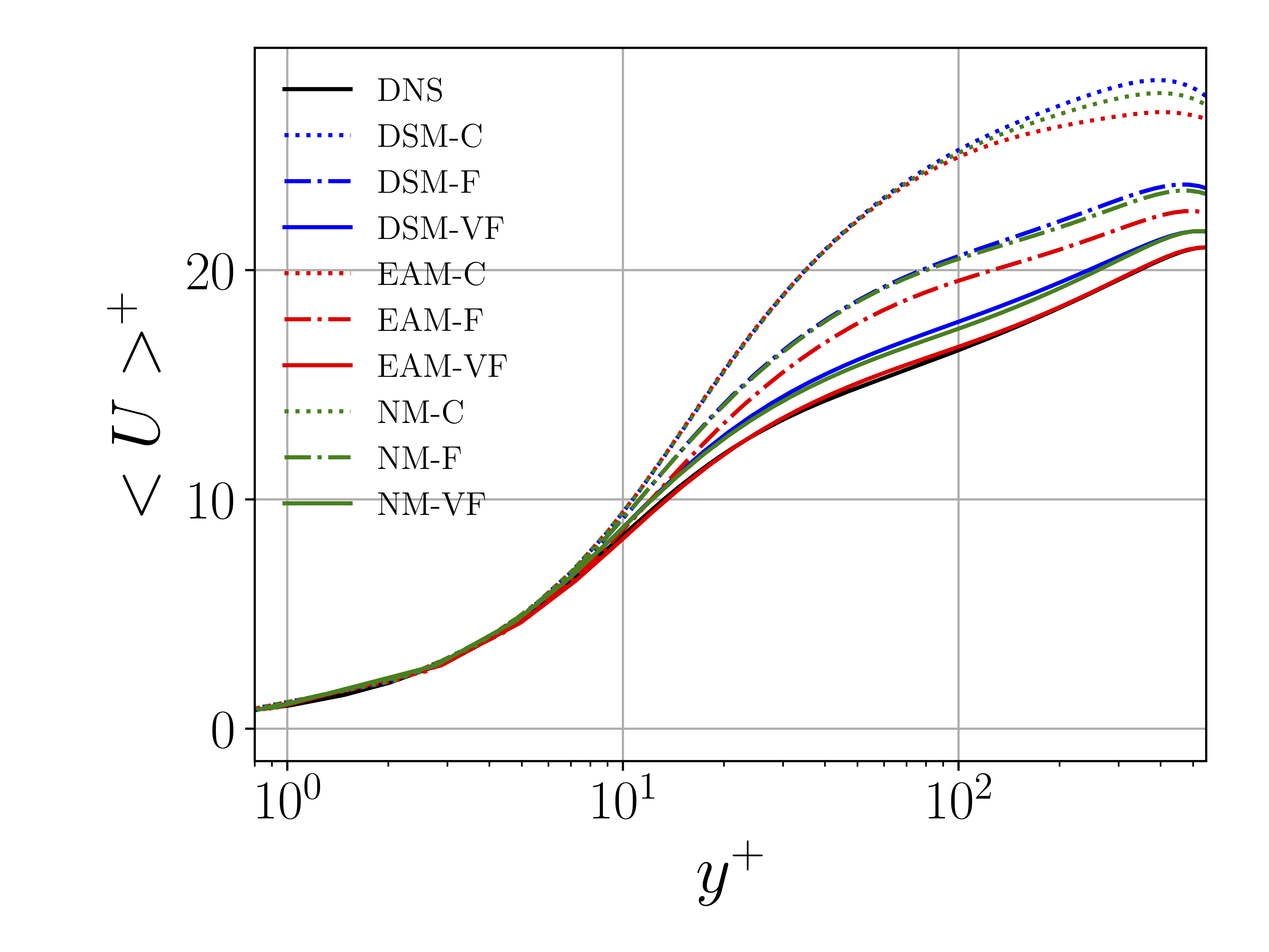
Explicit Algebraic modelling for hybrid RANS-LES computations
A new anisotropy-resolving hybrid RANS-LES model is formulated, where Explicit Algebraic Reynolds stress modelling (Wallin & Johansson, IJHFF 2002) is applied in both RANS and LES formulations. Introduced by Shur et al. (IJHFF 2008), IDDES methodology has an extended RANS-LES switching function which enables wall-modelled LES for sufficiently fine meshes. The novel EARSM-IDDES is an extension of the k-omega SST IDDES (Gritskevich et al., FTAC 2012), where the RANS modelling part of the EARSM-IDDES allows for a reasonable estimation of flows characterised by swirl, curvature and three-dimensional effects. Differently from the Explicit Algebraic SGS model where the SGS kinetic energy was estimated by a dynamic procedure (Marstorp et al., JFM 2009; Montecchia M., PhD thesis, 2019) the LES part of EARSM-IDDES employs the k-omega transport model equations for the evaluation of subgrid-scale kinetic energy.
In turbulent plane channel flows, EARSM is found to substantially reduce the RANS-LES transitional region, resulting in a substantially larger amount of resolved turbulence near the wall. This can be of importance in mitigating the so called grey-area problems resulting in a more rapid transition to resolved turbulence in more complex cases.
In periodic hill channel flows, the EARSM-IDDES has been tested on different grids with less than half million grid points. For both the Reynolds numbers, the skin friction is reasonably predicted. The EARSM gives a better estimation of the reattachment and is found to perform better than k-omega SST IDDES for the highest Reynolds number with a more pronounced separation between RANS and LES regions.
In 3-D diffuser flow, the friction coefficient is better predicted by using EARSM-IDDES and both the grids, the largest improvements are visible towards the inlet duct.
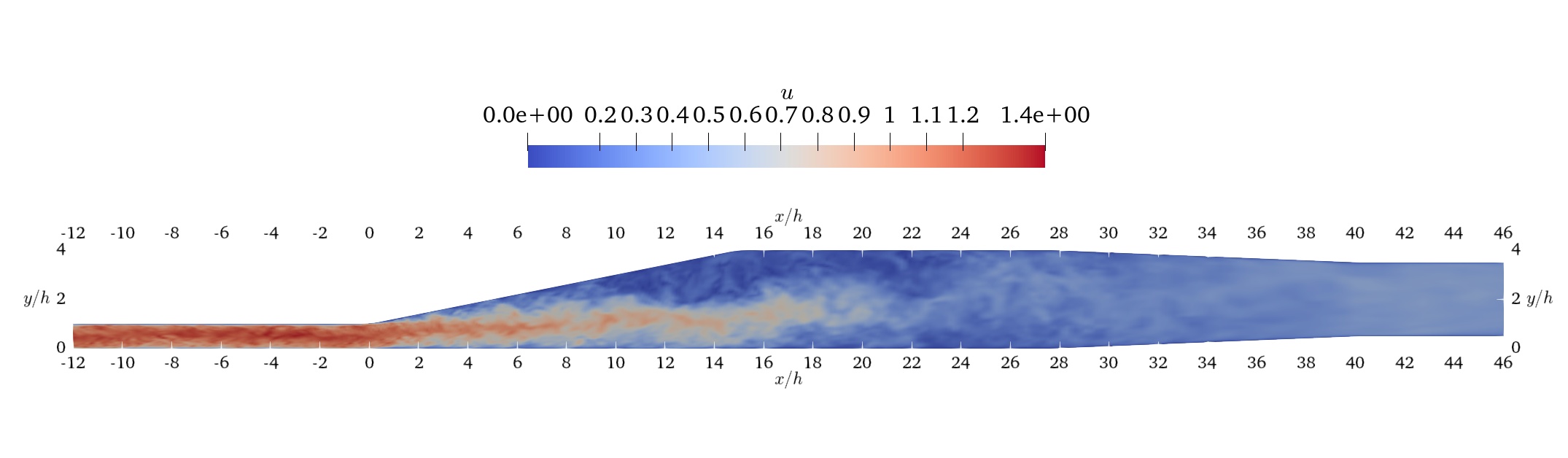
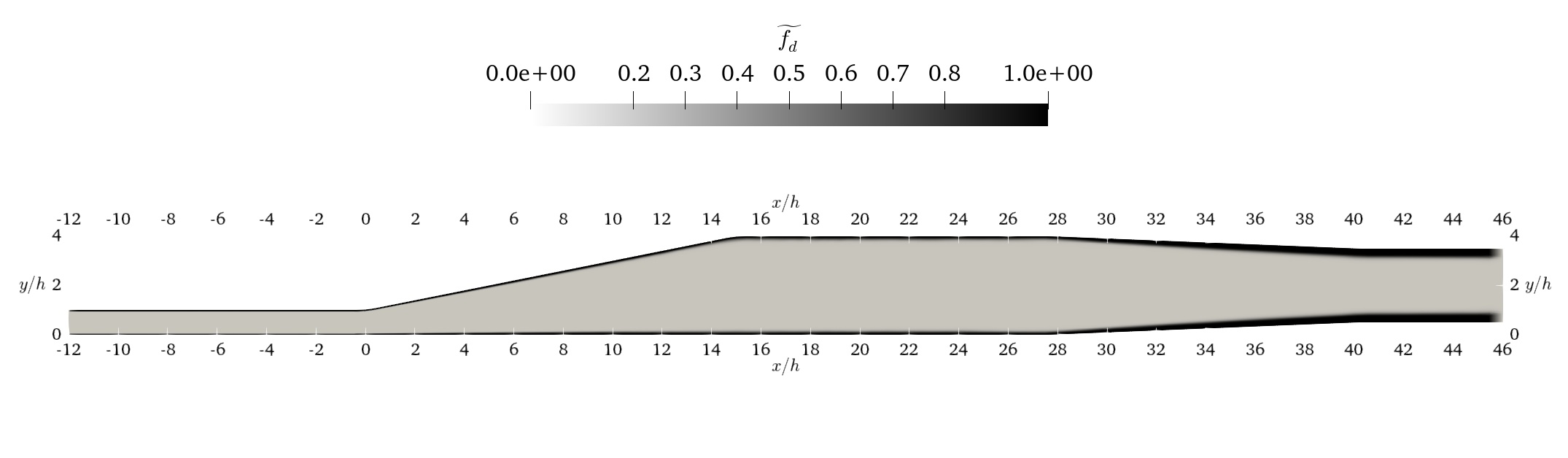
UHURA: Unsteady High-Lift Aerodynamics – Unsteady RANS Validation
High-lift devices of aircraft that increase the lift produced by the wing can reduce the speed and distance required for a safe take-off and landing and allow the use of a more efficient wing in the cruising phase of flight. The introduction of a Krüger flap enables the use of laminar wings for reduced environmental impact. The project UHURA investigates the unsteady flow behaviour around such Krüger flap during the deployment and retraction to gain an advanced understanding of critical aerodynamic features. One of the main goals of the project is to develop an accelerated CFD method that can accurately capture both the slow dynamics of mechanical components and the rapid turbulence scales. Within this frame, scripted meshing, moving/deforming mesh, fluid-structure interaction (FSI) interpolation scheme, and different URANS and hybrid RANS/LES models have been considered. For the transient turbulence modelling, the Delayed Detached Eddy Simulation (DDES) with the multigrid method and line-implicit acceleration has been proved to have a promising capability to maintain the prediction accuracy and computational time efficiency. More details about the project can be found here.